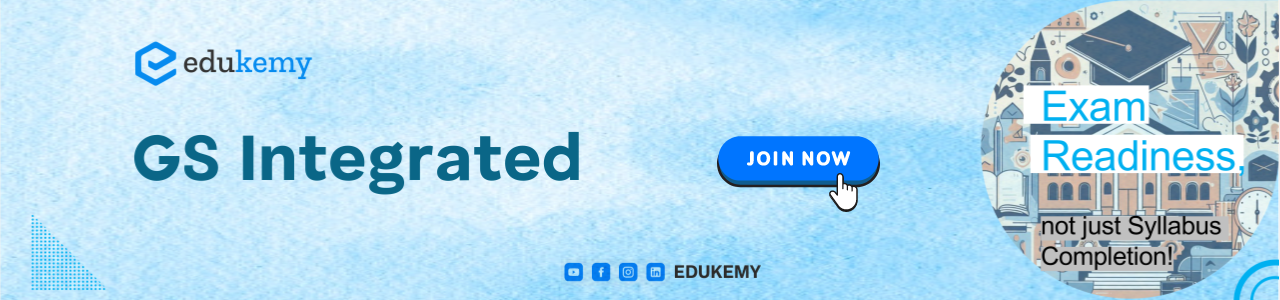
Nuclear energy is derived from the nucleus of atoms, predominantly through nuclear fission or fusion reactions, yielding substantial heat that can be converted into electricity. In India, nuclear power constitutes approximately 2% of the nation’s total electricity output, positioning it as the fifth-largest electricity provider in the country. Presently, India operates a network of seven power plants housing more than 22 nuclear reactors, collectively generating 6,780 MW of nuclear energy.
Contents
- 1 Nuclear Fusion
- 2 Nuclear Fission
- 3 Nuclear Energy
- 3.1 Nuclear Energy Landscape
- 3.2 National Strategy for Rapid Expansion of Nuclear Energy
- 3.2.1 Expansion of Pressurized Heavy Water Reactors (PHWRs):
- 3.2.2 Integration of Small Modular Reactors (SMRs) and Coal Plant Replacement:
- 3.2.3 Captive Units Catering to Industries:
- 3.2.4 High-Temperature Reactor for Hydrogen Production:
- 3.2.5 Thorium Energy Advancement:
- 3.2.6 International Collaboration:
- 3.3 Non-energy Applications of Nuclear Energy
- 4 FAQs
- 5 In case you still have your doubts, contact us on 9811333901.
Key Points on Nuclear Energy in India:
- Pioneering Indigenous Nuclear Technology: India stands out among developing nations as the sole producer of electricity utilizing domestically developed, demonstrated, and deployed nuclear reactors.
- Significant Contribution to India’s Energy Mix: Nuclear energy ranks fifth among India’s sources of electricity, contributing significantly to the nation’s power grid. Globally, India stands third in electricity production, generating 1207 TWh of electricity through nuclear means.
- Nuclear Infrastructure and Capacity: India holds the seventh position worldwide in terms of the number of nuclear reactors, boasting over 23 reactors spread across seven power plants nationwide. These reactors collectively produce 6780 MW of nuclear power.
- Future Expansion Goals: India aspires to elevate its atomic power contribution from the current 3.2% to 5% by the year 2031, reflecting the country’s commitment to enhancing its nuclear energy capacity and diversifying its energy portfolio.
Importance of Nuclear Energy
- Transitioning away from Fossil Fuels: Nuclear energy offers a viable solution to mitigate the adverse effects of fossil fuels, which are the primary contributors to global warming. Shifting focus to nuclear energy can help reduce dependency on fossil fuels.
- Achieving Net Zero Targets: There exists a global consensus to achieve net zero emissions between 2045 and 2070. India, in alignment with this goal, has pledged to become carbon neutral by 2070. Nuclear energy plays a pivotal role in this transition by facilitating the transformation of energy systems and adopting new technologies.
- Addressing Energy Needs: India’s energy requirements necessitate a minimum of 2400 kilogram oil equivalent energy consumption per capita annually. Efficient utilization of energy, including nuclear power, can significantly improve energy consumption to around 1400 kg per capita.
- Environmental Friendliness: Nuclear power serves as a clean and eco-friendly source of base load electricity generation, operating 24 x 7 without emitting harmful greenhouse gases like CO2, thus combating climate change.
- Enhancing Energy Security: Nuclear energy offers long-term energy security in a sustainable manner, reducing reliance on external energy sources and bolstering national energy autonomy.
- Promoting Sustainable Development: Embracing nuclear energy aligns with Sustainable Development Goal 7, which aims to ensure universal access to affordable, reliable, sustainable, and modern energy for all, thereby contributing to holistic and sustainable development.
Nuclear Fusion
Nuclear fusion stands as the most potent reaction observable in our universe, serving as the fundamental energy source for the Sun and other stars. The comprehension of nuclear fusion’s physics began to crystallize in the 1920s, with Arthur Eddington proposing that stars derive their energy from the fusion of hydrogen into helium.
Key Aspects of Nuclear Fusion:
- Fusion Process: Nuclear fusion involves the merging of two light atomic nuclei to form a heavier nucleus.
- Deuterium-Tritium (DT) Fusion Reaction: The fusion of deuterium and tritium generates a neutron and a helium nucleus. DT reactions are of particular interest to researchers due to their substantial energy output and the ability to occur at lower temperatures compared to other fusion elements.
- Thermonuclear Fusion: Fusion reactions take place within a plasma state—a hot, charged gas comprising positive and negative ions (free-moving electrons). This state exhibits distinct properties from solids, liquids, or conventional gases.
- Conditions for Fusion: To facilitate fusion, the protons or heavier nuclei of reactants must be brought close enough to overcome electrostatic repulsion, allowing the nuclear strong force to promote fusion. This necessitates heating the nuclei to exceptionally high thermonuclear temperatures, typically around 100 million degrees Celsius for deuterium and tritium nuclei.
- Energy Release: Fusion reactions liberate significantly more energy compared to chemical reactions, owing to the far greater binding energy that holds nucleons together.
Applications of Nuclear Fusion:
- Fusion Energy: Fusion reactors have the potential to harness fusion reactions to produce clean energy. The energy radiated by stars, including our Sun, derives from hydrogen fusion to form helium.
- Fusion Weapons: Nuclear fusion has been instrumental in the development of thermonuclear bombs (Hydrogen bombs), achieved through the utilization of small-scale fission bombs.
Advantages of Nuclear Fusion:
- Abundant Energy: Controlled fusion reactions yield substantially more energy compared to conventional methods such as coal, oil, or gas combustion, as well as traditional nuclear fission reactions.
- Zero GHG Emissions: Unlike fossil fuel combustion, nuclear fusion reactions, including fusion, do not release harmful substances like carbon dioxide or other greenhouse gases into the atmosphere.
- Minimal Radioactive Waste: While fusion neutrons can induce radioactivity, strategic material choices, such as proton–boron solutions, can minimize the production of long-lived radioactive waste.
- Limited Proliferation Risk: Initiating fusion reactions requires significant technical infrastructure and does not involve fissile materials like uranium or plutonium, thus mitigating the risk of proliferation.
Challenges Associated with Nuclear Fusion:
- High-Temperature Requirement: Achieving nuclear fusion on Earth necessitates temperatures reaching hundreds of millions of degrees Celsius, presenting a formidable technical obstacle.
- Plasma Stability: Fusion reactions occur within a plasma state, posing challenges in confining plasma within a reactor due to its inherent instability.
- Engineering Hurdles: Developing materials capable of withstanding fusion conditions for extended periods, including neutron damage, remains a significant engineering challenge. Currently, there is no facility available for comprehensive testing of fusion materials.
Global Efforts in Nuclear Fusion Research
- Tokamak Technology Development: Numerous countries, including India, are actively engaged in advancing Tokamak technology, a key component in nuclear fusion research.
- International Thermonuclear Experimental Reactor (ITER) Project: Established in France in 2007, ITER stands as the world’s largest international fusion facility. Its primary objective is to demonstrate the scientific and technological feasibility of fusion energy production. The project aims to achieve a fusion power gain of at least ten and to generate 500 Megawatts (MW) of fusion power. Member nations participating in ITER include China, the European Union, India, Japan, South Korea, Russia, and the United States.
- International Fusion Materials Irradiation Facility (IFMIF): IFMIF is a collaborative effort between Europe and Japan, with plans for construction in Japan. This facility is designed to support nuclear fusion research by providing a platform for the irradiation of fusion materials.
Nuclear Fission
Nuclear fission, a pivotal process in nuclear physics, involves the division of the nucleus of a heavy atom into two or more lighter atoms when bombarded by a neutron. This process yields a substantial amount of energy, accompanied by the emission of two or more neutrons. The initial evidence of nuclear fission was presented by German scientists Hahn and Strassman in 1938.
Process of Nuclear Fission
Nuclear fission initiates when a neutron collides with a uranium atom, resulting in the atom’s splitting and the release of significant energy in the form of heat and radiation. For instance, each uranium-235 (U-235) atom consists of 92 protons and 143 neutrons, totaling 235 particles. The internal structure of uranium-235 is inherently unstable. Upon absorbing an additional neutron, a U-235 nucleus promptly divides into two parts, known as fission.
Applications:
- Fission Reactors: Controlled nuclear fission is harnessed in nuclear reactors to generate clean electricity.
- Fission Bombs: Atomic bombs exploit uncontrolled fission reactions for devastating effects.
Harnessing Nuclear Fission Energy
Nuclear reactors serve as platforms for tapping into the vast potential of fission reactions by regulating the chain reaction through the use of moderators.
Chain Reaction
Fission chain reactions arise from interactions between neutrons and fissile isotopes like 235U. When a uranium atom undergoes fission, it releases additional neutrons, which then collide with other uranium atoms, perpetuating the process indefinitely. This continuous reaction constitutes a nuclear chain reaction, sustaining itself in a cycle. In nuclear power plants, this reaction is meticulously controlled to produce the desired amount of heat.
Advantages of Nuclear Fission
- Cost-Effectiveness: Nuclear fission presents a financially viable and readily available energy solution, constituting over 10% of the world’s electricity consumption.
- Efficiency and Affordability: Once operational, nuclear power stands out as one of the most efficient and economically viable energy sources.
- Environmentally Friendly: Unlike conventional power generation methods, nuclear fission does not contribute to global warming or air pollution, making it a clean technology solution.
- Reliability: Nuclear fission plants boast operational lifespans of 30-50 years, with uranium reserves worldwide capable of sustaining operations for another century, ensuring uninterrupted energy production regardless of weather conditions.
Disadvantages of Nuclear Fission
- Hazardous Waste: While nuclear fission generates clean energy, the disposal of radioactive waste poses significant environmental risks and challenges.
- Proliferation of Nuclear Weapons: The technology behind nuclear fission can be manipulated to develop destructive weapons like nuclear bombs, presenting a global security threat.
- Risk of Accidents: Catastrophic nuclear accidents like Chernobyl and Fukushima underscore the potential dangers associated with nuclear fission power plants.
- Radiation Hazards: The radiation emitted by nuclear fission processes can be harmful or even fatal to humans and wildlife, particularly in high doses.
- High Initial Investment: The construction of nuclear power plants requires substantial financial investment due to the intricate technologies and stringent safety protocols involved.
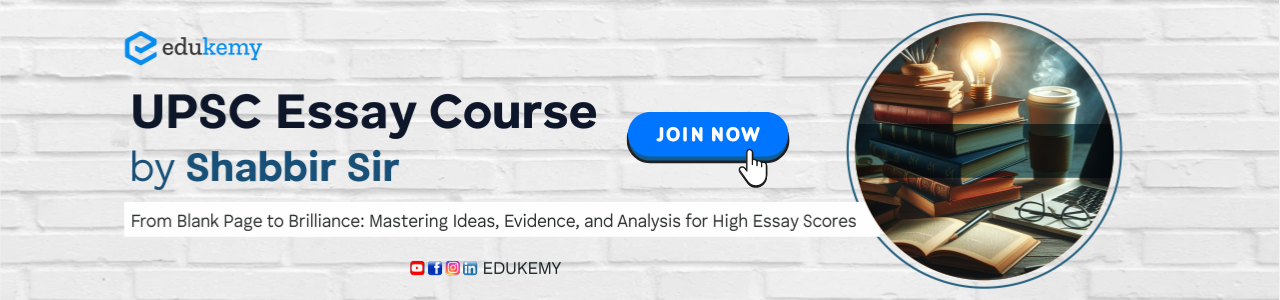
Nuclear Energy
Nuclear policy denotes a framework of directives, principles, and tactics that a nation adopts concerning its nuclear weapons arsenal and nuclear energy initiatives.
It is imperative for overseeing the progression, deployment, and potential utilization of nuclear weapons while ensuring strict regulation to deter proliferation and prevent accidental or deliberate usage. Nuclear policy extends to the prudent administration and oversight of nuclear energy endeavors. While nuclear energy holds promise as a clean and dependable energy source, it concurrently entails inherent risks, including the prospect of nuclear accidents and the dissemination of nuclear weapons technology.
Nuclear Energy Landscape
India’s journey in nuclear energy commenced with a vision to harness atomic power for peaceful purposes, aimed at enhancing people’s lives and achieving energy self-sufficiency.
The nuclear program, which initially took modest steps, has evolved into a vast and multifaceted initiative, comprising 63 organizations under the Department of Atomic Energy (DAE).
These organizations engage in a diverse array of activities, including research and development in nuclear sciences, exploration and mining of radioisotopes, nuclear energy deployment, application of nuclear technology in agriculture and medicine, among others.
India’s nuclear program is structured around a distinctive three-stage strategy and associated technologies, designed to optimize the utilization of its nuclear resources, which include modest Uranium reserves and abundant Thorium deposits. This strategy entails a closed fuel cycle, where spent fuel from one stage is reprocessed to generate fuel for the subsequent stage.
The commercialization of nuclear power in the first stage, employing Pressurized Heavy Water Reactors (PHWRs) and imported Light Water Reactors (LWRs), is overseen by the Nuclear Power Corporation of India Limited (NPCIL). The second stage, featuring Fast Breeder Reactors (FBRs), is managed by Bharatiya Nabhikiya Vidyut Nigam Limited (BHAVINI), both of which are wholly owned by the central government as per statutory provisions.
STAGE 1: Pressurized Heavy Water Reactor
Utilizes natural UO2 as fuel matrix. Heavy water serves as moderator and coolant.
STAGE 2: Fast Breeder Reactor
The second phase involves the use of Pu-239, derived from the first stage reactor, as the core fuel in fast breeder reactors (FBRs).
STAGE 3: Breeder Reactor
India’s third phase focuses on breeder reactors using U-233 fuel, leveraging the nation’s abundant thorium reserves.
Research Reactors:
The DHRUVA Reactor at BARC, designed and commissioned by Indian scientists and engineers, utilizes natural U as fuel and heavy water as moderator and coolant. It has enabled India to achieve self-sufficiency in radioisotope production. Kamini, a 30 kWt reactor at the Indira Gandhi Centre for Atomic Research in Kalpakkam, operational since October 1996, facilitates neutron radiography and represents a significant stride in leveraging India’s vast thorium reserves. It stands as the world’s sole operating reactor using U-233 fuel.
National Strategy for Rapid Expansion of Nuclear Energy
Expansion of Pressurized Heavy Water Reactors (PHWRs):
- The focal point lies in expanding the Indigenous 700 MWe PHWRs, already operational, to bolster base load electrical capacity.
- Ongoing construction of fifteen additional units underscores the commitment to fleet expansion.
- A collaborative approach involving various Public Sector Undertakings (PSUs) alongside NPCIL should be explored for multiple fleet implementations.
Integration of Small Modular Reactors (SMRs) and Coal Plant Replacement:
- Indigenous development of Small Modular Reactors (SMRs) is prioritized for sites vacated by retiring coal plants in the forthcoming years.
- Importing such units would incur unaffordable electricity production costs, hence necessitating domestic production.
- Key stakeholders like NTPC, with a significant stake in coal plants, should be engaged in this transition alongside additional industrial partners.
Captive Units Catering to Industries:
- The deployment of 220 MWe PHWR units can serve as partially owned captive units, catering to energy-intensive industries like metals, chemicals, and fertilizers.
- The Advanced Heavy Water Reactor (AHWR300-LEU) from BARC could potentially serve this function post-prototype demonstration.
High-Temperature Reactor for Hydrogen Production:
- The development of high-temperature reactors for direct hydrogen production is envisaged to facilitate cost-effective green hydrogen production.
- This approach alleviates pressure on excessive electrification of the energy system, offering a viable alternative.
Thorium Energy Advancement:
- Accelerated development of second and third stage nuclear-power programmes aims to harness the untapped potential of thorium energy.
- Leveraging Bhabha Atomic Research Centre’s expertise is pivotal in driving this initiative forward.
International Collaboration:
- India’s globally competitive PHWRs, coupled with advancements like Thorium-High Assay Low Enriched Uranium (HALEU) fuel, present a compelling case for international cooperation.
- Pilot initiatives for major international collaborations could effectively address climate change challenges on a global scale, positioning India as a proactive contributor to sustainable energy solutions.
Non-energy Applications of Nuclear Energy
Nuclear energy holds immense potential to address global energy and water security challenges through non-electric applications, including seawater desalination, hydrogen production, district heating, and various industrial processes. The International Atomic Energy Agency (IAEA) actively promotes and facilitates the exploration of new and emerging applications of nuclear technologies.
Non-electric applications fueled by nuclear energy offer sustainable solutions to present and future energy needs. Interest in leveraging nuclear energy for
- seawater desalination
- hydrogen production
- district heating
- industrial processes
Nuclear desalination emerges as a viable solution to address the escalating demand for potable water, especially in arid and semi-arid regions grappling with severe water scarcity. It also proves invaluable in optimizing water management for nuclear power plants, ensuring consistent water supply throughout construction, operation, and maintenance phases.
Hydrogen, a vital resource lacking natural reservoirs, serves as a pivotal component in various industrial sectors. Recognized as an eco-friendly energy carrier, hydrogen holds promise as a clean fuel for transportation, contributing minimally to global warming. Nuclear hydrogen production technologies exhibit significant potential, boasting advantages over alternative sources. High-temperature nuclear reactors offer efficient electricity input to hydrogen production, enhancing economic viability while reducing carbon footprint.
Industrial applications and nuclear cogeneration involve synergizing nuclear power plants with diverse systems and processes. Beyond desalination and hydrogen production, nuclear-generated heat finds application in cooling, heating, and other industrial processes, amplifying its utility.
The IAEA plays a pivotal role in fostering the development of new nuclear applications, particularly in co-generation and heat utilization, including seawater desalination. It facilitates information exchange, publishes technical and economic insights, collaborates with Member States on research programs, and organizes technical forums to advance non-electric applications of nuclear energy. The Agency also offers software tools empowering decision-makers with insights into the feasibility of nuclear-driven non-electric applications.
FAQs
1. What is nuclear energy?
Nuclear energy is the energy released during nuclear reactions, either through nuclear fission or nuclear fusion processes. In nuclear fission, the nucleus of an atom splits into smaller parts, releasing a large amount of energy. In nuclear fusion, atomic nuclei combine to form a heavier nucleus, also releasing a substantial amount of energy.
2. What is radioactivity?
Radioactivity is the spontaneous emission of radiation from the nucleus of an unstable atom. This emission occurs when the nucleus undergoes a decay process to achieve a more stable configuration. The emitted radiation can take the form of alpha particles, beta particles, or gamma rays.
3. How does nuclear fission work?
Nuclear fission involves the splitting of a heavy nucleus, typically that of uranium or plutonium, into lighter nuclei. When a neutron collides with a heavy nucleus, it can cause it to become unstable and split into two or more smaller nuclei, releasing additional neutrons and a significant amount of energy in the process. This energy is utilized in nuclear reactors to generate electricity.
4. What is nuclear fusion?
Nuclear fusion is a process in which atomic nuclei combine to form a heavier nucleus, releasing a large amount of energy in the process. Unlike nuclear fission, which involves the splitting of atoms, nuclear fusion involves the fusion of lighter nuclei, such as isotopes of hydrogen (e.g., deuterium and tritium). Nuclear fusion powers the sun and other stars and has the potential to provide a nearly limitless source of clean energy on Earth.
5. What are the non-energy applications of nuclear energy?
Nuclear energy finds various non-energy applications, including medical uses such as cancer treatment (radiotherapy), diagnostic imaging (e.g., X-rays, PET scans), and sterilization of medical equipment. It is also used in agriculture for food irradiation to extend shelf life and control pests. Additionally, nuclear techniques are employed in environmental monitoring, archaeology, and materials science.
Bonus: What are the challenges associated with nuclear energy?
Despite its benefits, nuclear energy poses several challenges, including the management and disposal of radioactive waste, the potential for accidents and their consequences (e.g., Chernobyl, Fukushima), proliferation of nuclear weapons, high initial capital costs for building nuclear power plants, and public perception concerns regarding safety and environmental impact. Additionally, the development of nuclear fusion as a practical energy source faces significant technical and engineering hurdles.
In case you still have your doubts, contact us on 9811333901.
For UPSC Prelims Resources, Click here
For Daily Updates and Study Material:
Join our Telegram Channel – Edukemy for IAS
- 1. Learn through Videos – here
- 2. Be Exam Ready by Practicing Daily MCQs – here
- 3. Daily Newsletter – Get all your Current Affairs Covered – here
- 4. Mains Answer Writing Practice – here