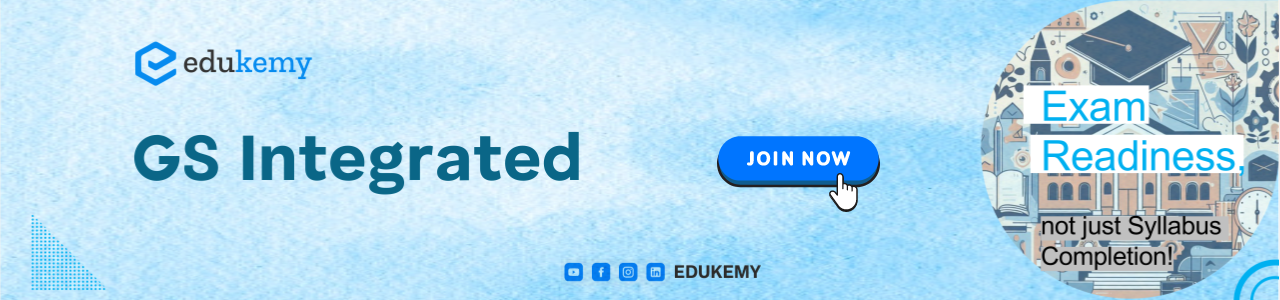
Renewable energy, often hailed as the beacon of sustainable development, encompasses a diverse array of sources that harness natural processes to generate power. Solar energy, deriving from the sun’s rays, has become synonymous with clean energy initiatives, employing photovoltaic cells to convert sunlight into electricity. Wind power, driven by the kinetic energy of moving air masses, propels turbines to produce electricity, making it a prevalent feature of modern renewable energy landscapes. Tidal and wave energy tap into the gravitational forces of the moon and the kinetic energy of ocean waves, respectively, offering promising avenues for coastal regions. Geothermal energy delves into the Earth’s heat reservoirs, utilizing steam or hot water to drive turbines and generate electricity. Fuel cells, operating through chemical reactions, offer portable and efficient power solutions, while ocean thermal energy conversion (OTEC) leverages temperature differentials in ocean water to produce electricity. Beyond these prominent sources, a multitude of other renewable energy options, from biomass to hydroelectricity, contribute to the global shift towards sustainable energy systems.
Renewable Energy
Renewable energy refers to energy derived from naturally replenished resources, such as solar energy, geothermal heat, wind, tides, water, and various forms of biomass. Unlike fossil fuels, renewable energy sources are continuously replenished and never depleted. They offer a sustainable and environmentally friendly alternative to traditional energy sources by emitting less pollution and being readily available locally.
Renewable Energy Status in India
India stands as a prominent player in the realm of renewable energy. As the world’s third-largest electricity consumer and producer of renewable energy, India has made significant strides in expanding its renewable energy capacity. In 2020, renewable energy accounted for 38% (136 GW out of 373 GW) of India’s total installed energy capacity.
According to the 2021 Renewable Energy Country Attractiveness Index (RECAI) by Ernst & Young (EY), India ranks third globally, following the United States and China, in terms of renewable energy attractiveness. In alignment with its commitments under the Paris Agreement, India aims to generate 50% of its total electricity from non-fossil fuel sources by 2030.
The government has set ambitious targets to achieve 175 GW of renewable energy capacity by 2022, with specific goals for solar, wind, biopower, and small hydropower. Additionally, India aims to reach 500 GW of installed electricity capacity from non-fossil fuel sources by 2030, as pledged at COP26.
India’s renewable energy landscape showcases significant achievements, with operational solar energy capacity reaching 89.22 GW as of September 2020. Notable solar parks like the Bhadla Solar Park in Rajasthan, Pavgada Solar Park in Karnataka, and Kurnool Solar Park in Andhra Pradesh underscore India’s commitment to solar energy development.
In wind power, India boasts a robust manufacturing base and ranks fourth globally in installed wind power capacity. Furthermore, India ranks fifth worldwide in installed hydroelectric power capacity, with significant potential for further development.
Despite progress in renewable energy, nuclear power remains a notable contributor to India’s energy mix, with 10 nuclear reactors under construction and 23 existing reactors in operation. However, nuclear power constitutes only a fraction of India’s total electricity generation, following coal, gas, hydroelectricity, and wind power.
As of April 2022, India’s installed renewable energy capacity stands at 158.12 GW, representing 39.43% of total installed power capacity. With solar, wind, hydro, and other renewable sources playing increasingly pivotal roles, India remains committed to advancing its renewable energy agenda to meet its growing energy demands while mitigating environmental impacts.
Advantages of Renewable Energy:
- Private-sector Opportunity: The renewable energy sector presents a lucrative $20 billion-per-year business opportunity, with a target of achieving 450 GW of renewable energy capacity by 2030, translating to an annual addition of 25-30 GW.Â
- Low Maintenance Costs: Renewable energy sources like solar offer minimal maintenance costs compared to traditional coal or oil-based thermal power plants, as they require no fuel procurement and have fewer moving parts.Â
- Government Incentives: Government incentives drive investment in renewable energy, fostering additional capacity expansion and resource utilization.Â
- Sustainability: Renewable energy’s cleaner profile benefits the environment by reducing pollution and associated health risks, promoting overall sustainability.Â
- Atmanirbhar Bharat: Private sector investments in renewable energy align with the government’s vision of self-reliance and stimulate job creation opportunities nationwide.Â
- Last-mile Connectivity: Decentralized renewable energy solutions enable cost-effective last-mile connectivity in remote areas, reducing transmission losses and enhancing energy access for citizens.Â
Disadvantages of Renewable Energy :Â
- Variability of Solar and Wind Energy: The intermittent nature of solar and wind energy poses challenges in availability and consistency, requiring supplementary energy sources to maintain base load requirements.Â
- Battery Storage Investment: To address renewable energy variability, substantial investments in large-capacity batteries are necessary, requiring government commitment to bolster investor confidence.Â
- Initial Investment for Large-scale Projects: Establishing large-scale renewable energy projects demands significant upfront investment, potentially deterring early-stage project development.Â
- Need for Domestic Manufacturing: Promoting Atmanirbhar Bharat necessitates building manufacturing capacity in India to reduce imports and foster job creation, particularly in the renewable energy sector.Â
Solar Energy
- Solar energy refers to the radiant light and heat emitted by the Sun, harnessed through various technologies for electricity generation, heating water, and architectural purposes.
- It is a significant renewable energy source, categorized as passive solar or active solar based on its capture and distribution methods.
- Active solar techniques include photovoltaic systems, concentrated solar power, and solar water heating, while passive solar methods involve building orientation, material selection, and natural air circulation.
- Solar energy’s abundance and affordability make it an attractive electricity source, surpassing fossil fuels in cost-effectiveness since 2021.
Photovoltaic (PV) Electricity:
- PV cells, composed of semiconductor layers, absorb sunlight photons, releasing electrons that create a voltage difference.
- Electrons flow through an external circuit, generating electricity when PV cell layers are connected to a load.
- Inverters convert generated power from direct current (DC) to alternating current (AC) for usage.
Solar-Thermal Electricity:Â
- Solar-Thermal Electricity employs mirrored collectors to reflect sunlight onto receivers, heating liquids to produce steam for electricity generation.
- Solar thermal power systems use reflectors to concentrate sunlight onto receivers, generating high-temperature heat for power production.
Solar Thermal Technology:
- Solar thermal technologies utilize mirrors and lenses to concentrate sunlight, absorbing heat for applications like water and building heating.
- Various types of solar thermal collectors include unglazed, transpired air, flat-plate, and evacuated tube collectors.
Concentrated Solar Power (CSP):
- CSP technologies focus sunlight to generate high-temperature heat, which drives conventional generators to produce electricity.
- Parabolic troughs and other collectors capture sunlight to heat fluids like synthetic oil or molten salt, powering turbines for electricity generation.
- Inverters convert generated DC power to AC for transmission and usage.
India’s Solar Energy Capacity:
- India holds the fifth position globally in terms of installed solar power capacity, following China, the United States, Japan, and Germany.
- As of December 2021, India boasts a cumulative solar installed capacity of 55 GW, representing approximately half of the nation’s renewable energy capacity (excluding large hydropower) and 14% of total power generation capacity.
- Grid-connected utility-scale projects constitute 77% of the total solar capacity, with grid-connected rooftop and off-grid projects contributing the remaining share.
India’s Grid-connected Rooftop Solar Programme:
- Launched in December 2015, India initiated the first phase of its grid-connected rooftop solar program to promote solar usage in residential, institutional, and social settings.
- The second phase, sanctioned in February 2019, aimed to achieve a cumulative rooftop solar capacity of 40 GW by 2022, supported by central financial assistance (CFA).
- As of November 2021, only 1.1 GW of the 4 GW target for the residential sector had been installed.
Wind Energy
Wind Farm:
- Wind farms are vast areas where numerous large wind turbines are grouped together to harness wind energy.
- These turbines, resembling super-tall windmills, capture the energy present in the wind.
- Wind farms can span hundreds of kilometers, with hundreds of turbines strategically placed across the landscape.
- The land surrounding the turbines can often be utilized for other purposes, such as traditional farming or conservation.
- Some wind farms are situated near bodies of water, capitalizing on the winds blowing across lakes or oceans.
Types of Wind Farms:
Onshore Wind Farms:
- Onshore wind farms are established on land to generate electricity using wind energy.
- They are typically located in areas with minimal conservation or habitat significance.
Advantages:Â
- Cost-effective: Onshore wind farms are generally cost-effective, facilitating large-scale installations.
- Reduced Voltage Drop: Due to shorter distances between turbines and consumers, voltage drop on cabling is minimized.
- Quick Setup: Wind turbines can be installed relatively quickly compared to other energy infrastructure.
Disadvantages:Â
- Visual Impact: Many individuals view onshore wind farms as visually intrusive on the landscape.
- Seasonal Energy Production: Wind speed variations and physical obstacles can result in seasonal energy production.
- Noise Pollution: Wind turbine noise can be comparable to that of a lawnmower, contributing to local noise pollution.
Offshore Wind Farms:
- Offshore wind power involves generating electricity from wind over open water, typically in oceans.
- These wind farms are established in regions with higher wind speeds, such as bodies of water.
Advantages:Â
- Increased Energy Production: Larger turbines offshore enable greater energy collection.
- Minimal Intrusion: Offshore farms are usually situated far from land, minimizing visual impact and allowing for larger installations.
- Higher Wind Speeds: Open sea areas generally experience higher wind speeds, enhancing energy generation.
- Environmental Impact: Offshore wind farms have minimal environmental impact, avoiding shipping channels, fishing areas, and sensitive habitats.
Disadvantages:Â
- Costly Infrastructure: Offshore wind farms are expensive to construct and maintain due to their remote locations and exposure to extreme weather.
- Marine Life Impact: The effects of offshore wind farms on marine life and bird populations are still under study.
- Property and Tourism Concerns: Offshore farms near coastlines may face local opposition due to potential impacts on property values and tourism.
Wind Energy Potential in India:
- Over recent years, India has experienced a significant expansion in its wind power generation capacity.
- Regions like the Southern, Western, and Northern parts of the country boast substantial wind power potential.
- From 2010 to 2020, the compound annual growth rates for wind generation and installed capacity stood at 11.39% and 8.78%, respectively.
- India’s vast 7,600 km coastline harbors the potential to generate 127 GW of offshore wind energy, as per the Ministry of New and Renewable Energy (MNRE).
- At a hub height of 100 meters, the National Institute of Wind Energy (NIWE) estimates the total wind energy potential to be 302 GW.
- States such as Andhra Pradesh, Gujarat, Karnataka, Madhya Pradesh, Maharashtra, Rajasthan, and Tamil Nadu collectively possess over 95% of commercially exploitable wind resources.
- The MNRE has set ambitious targets of installing 5 GW of offshore wind by 2022 and 30 GW by 2030.
Installed Wind Energy Capacity:
- India’s wind energy sector has seen remarkable progress, driven by its indigenous wind energy industry.
- This growth has fostered a robust ecosystem, enhanced project operating capabilities, and established a manufacturing base producing approximately 10,000 megawatts annually.
- With a cumulative installed capacity of 39.25 GW by March 31, 2021, India ranks fourth globally in wind installed capacity, generating around 60.149 billion units in 2020-21.
- The government has deployed over 800 wind-monitoring stations nationwide and disseminated wind potential maps at varying heights above ground level through NIWE.
- Private sector investment in wind power projects is actively encouraged across India through incentives such as Accelerated Depreciation and concessional custom duty exemptions.
- The Generation Based Incentive (GBI) Scheme, available for wind projects completed before March 31, 2017, further incentivizes investment in this sector.
Hydropower
Hydropower involves utilizing the natural force of water to generate electricity, tapping into its kinetic energy. Hydroelectric systems capture this energy and convert it into power through the use of turbines and generators. Renowned for its renewable nature, hydropower relies on the replenishment of water by the sun, making it a sustainable and eco-friendly energy source that does not emit greenhouse gases. China leads the world in hydropower generation, followed by Canada, Brazil, and the United States, highlighting its global significance. With its abundance and sustainability, hydropower is poised to remain a dominant energy resource for years to come.
Diverse Types of Hydropower Plants
Impoundment Hydropower Plant:
This prevalent type of hydroelectric plant utilizes a dam to create a reservoir, storing river water. The released water spins turbines, activating generators to produce electricity. Beyond power generation, impoundment plants serve various purposes including flood control, recreation, and environmental preservation.
Diversion Hydropower Plant:
Also known as run-of-river plants, diversion plants divert a significant portion of river flow into pipelines or tunnels. Water drives turbines before returning to the river’s main channel, generating electricity without installing turbines in the river.
Pumped Storage Hydropower Plant:
Pumped-storage hydroelectricity involves transferring water from a lower to a higher reservoir to store gravitational potential energy. During peak demand, stored water is released through turbines to generate electricity, leveraging surplus off-peak electric power to boost revenue.
Small Hydro Power (SHP):
Projects with less than 25 MW capacity are classified as small hydro power, mainly run-of-river with minimal water storage. They have lesser environmental impact compared to larger hydro projects and can independently serve isolated rural communities.
India holds vast potential in small hydro power, with over 5,400 sites identified boasting a total capacity of 19,750 MW. Projects in Himalayan states and irrigation canals present significant opportunities for small hydro development. The Ministry of New and Renewable Energy is actively supporting both public and private sector initiatives, aiming to harness at least half of the current potential within the next decade.
Hydropower Status in India:Â
India boasts the fifth-largest installed hydroelectric power capacity globally, signifying its significant contribution to renewable energy. India’s utility-scale hydroelectric capacity stood at 46,000 MW, constituting 12.3 percent of the nation’s total utility power production capacity.
In addition to major hydroelectric units, India has installed smaller hydroelectric power units totaling 4,683 MW, contributing 1.3 percent to the total utility power generation capacity. With a load factor of 60%, India’s hydroelectric power potential is estimated at an impressive 148,700 MW.
In the fiscal year 2019–20, India generated 156 TWh of hydroelectric electricity (excluding small hydro), operating at an average capacity factor of 38.71 percent. Notably, the Darjeeling and Shivanasamudram hydroelectric power stations, operational since 1898 and 1902 respectively, represent India’s early foray into hydroelectric power, underscoring its pioneering role in Asia’s hydroelectric landscape.
Ocean Thermal Energy Conversion (OTEC)
Ocean Thermal Energy Conversion (OTEC) represents an innovative marine renewable energy technology that taps into the vast solar energy reservoirs harbored by the oceans.
The principle behind OTEC lies in the inherent temperature gradient existing within the ocean’s depths, where surface waters are warmed by the sun far more than the deeper layers. This thermal contrast serves as the foundation for OTEC’s operation.
By utilizing a working fluid with a low boiling point, such as ammonia, OTEC systems leverage the warm surface waters, typically around 25°C (77°F), to vaporize the fluid. The resulting vapor drives turbines connected to generators, thus generating electricity. Subsequently, cold seawater from the deeper layers, with temperatures hovering around 5°C (41°F), facilitates the condensation of the vapor back into a liquid state, completing the cycle for continuous power generation.
The efficacy of OTEC systems heavily depends on the temperature differential between surface and deep ocean waters. The greater the temperature gradient, the more efficient the energy conversion process. Consequently, OTEC technology finds practical applicability primarily in tropical regions characterized by substantial year-round temperature disparities of at least 20 degrees Celsius (36 degrees Fahrenheit).
Types of Ocean Thermal Energy Conversion Systems
Closed System:
In the closed OTEC system, a low-boiling-point working fluid is circulated to power turbines for electricity generation. Warm seawater drawn from the ocean’s surface initiates the evaporation of the fluid in a heat exchanger, propelling turbines within the generator. Upon contact with cold deep-sea water, the vapor condenses back into a liquid, facilitating its reuse within the closed loop system.
Open System:
Conversely, the open cycle OTEC harnesses power directly from the warm surface waters. The heated seawater undergoes boiling within a low-pressure chamber, courtesy of reduced boiling points induced by pressure differentials. The resulting steam propels turbines, driving electric generators. Notably, the open system yields desalinated water in the form of steam, free from contaminants, suitable for diverse applications spanning domestic, industrial, and agricultural sectors.
Ocean Energy:
A. Wave Energy
Wave energy presents an immense potential for renewable energy generation, with estimates suggesting a global wave resource of up to 2 terawatts (TW) – equivalent to the world’s total electricity consumption.
Harnessing wave energy could potentially meet over 40% of the world’s energy needs, a figure comparable to the output of more than 800 nuclear power plants.
Wave energy, derived from the transmission and aggregation of energy by ocean surface waves, holds promise for various applications, including electricity generation, water desalination, and water pumping.
As wind interacts with the water surface, it imbues waves with substantial energy, making them potent renewable resources. The energy output of waves is determined by factors such as wave speed, height, wavelength, and water density.
Despite its vast potential, wave energy remains challenging to harness effectively, resulting in limited implementation of wave energy plants worldwide.
B. Tidal Energy
Tidal energy, arising from the gravitational interplay between the Earth, the sun, and the moon, offers another avenue for sustainable power generation. While global tidal energy potential is estimated at 3,000 gigawatts, the usable energy for power generation through tidal barrages ranges from 120 to 400 gigawatts, depending on geographical location and conversion efficiency.
The natural ebb and flow of tides serve as the foundation for tidal energy generation, with barrages creating reservoirs behind them to harness tidal waters through turbines.
Tidal energy represents kinetic energy derived from the movement of tides and oceans, with tidal generators converting tidal flows into electricity through turbine propulsion.
Sites with greater tidal variation and higher tidal current velocities boast enhanced potential for tidal electricity generation, making tidal energy a promising component of the renewable energy landscape.
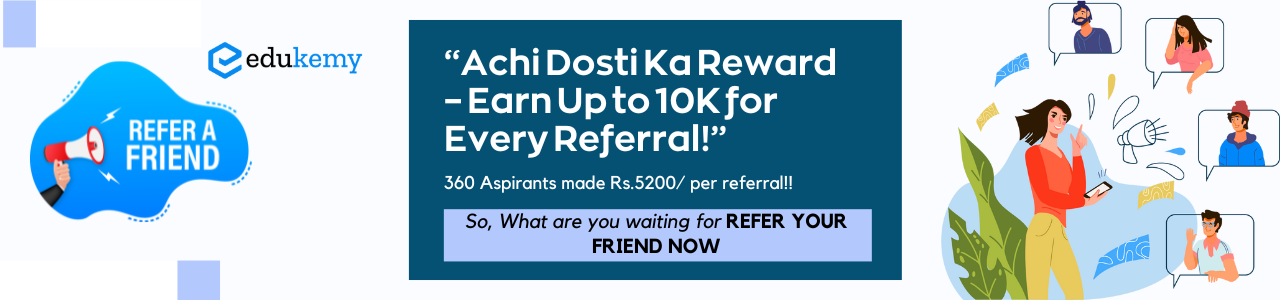
Waste-to-energy
Waste-to-energy (WtE) embodies the innovative process of converting waste into valuable energy resources, including electricity, heat, and fuels. Distinguished from traditional incinerators, modern waste-to-energy facilities prioritize the removal of hazardous and recyclable materials before transforming waste into energy.
While municipal solid waste constitutes the primary source for most waste-to-energy plants, certain facilities also process industrial or hazardous waste streams. Modern waste-to-energy plants emphasize waste segregation prior to combustion, facilitating recycling alongside energy generation.
Understanding Waste-to-Energy Plants:
Waste-to-energy (WTE) plants serve as pivotal facilities that harness waste for power generation, commonly known as trash-to-energy or municipal waste incineration plants.
Across urban India, various WTE facilities are emerging, employing diverse technologies such as incineration, RDF-based combustion, and advanced conversion techniques like pyrolysis and gasification.
Amidst the proliferation of WTE plants, concerns persist regarding technological intricacies, potential impacts on air quality, and public health implications. Additionally, questions surrounding the economic viability of these plants underscore ongoing debates.
Incineration-based waste-to-energy plants execute complete combustion of municipal solid waste, yielding residual ash as a byproduct.
Waste-to-Energy Techniques:
- Incineration/Combustion
Incineration involves the combustion of waste in large furnaces at elevated temperatures, transforming organic materials into bottom ash, flue gases, particles, and heat, which are harnessed to generate electricity.
This method significantly reduces trash volume by 95-96%, offering an effective landfill reduction strategy. In countries like Japan, where land availability is limited, incineration serves as a prevalent waste management solution.
Early incinerators lacked material separation systems, leading to the combustion of toxic, bulky, or recyclable items. Inadequate gas cleaning and combustion control posed health risks to plant personnel and the environment, often without power generation capabilities.
- Pyrolysis/Gasification
Pyrolysis entails the thermal decomposition of organic substances, generating gas molecules in the absence of oxygen. Gasification occurs when organic matter undergoes partial combustion in the presence of limited oxygen, resulting in syngas production.
Syngas, comprising carbon monoxide, hydrogen, hydrocarbons, carbon dioxide, and nitrogen, must undergo purification before utilization. It is subsequently employed to generate power in internal combustion engine generating sets or turbines.
- Cogeneration
Cogeneration, or combined heat and power (CHP), involves the sequential generation of mechanical and thermal energy from a single primary energy source. Mechanical energy powers alternators or rotating equipment, while thermal energy supports various processes.
Cogeneration optimizes energy utilization by repurposing otherwise wasted heat, such as exhaust from manufacturing plants, to provide additional energy benefits like building heat or power. This approach enhances cost-effectiveness and environmental sustainability by reducing reliance on polluting fossil fuels.
- Anaerobic Digestion
Anaerobic digestion is a microbial process that decomposes organic matter in the absence of oxygen, converting materials like animal dung, wastewater biosolids, and food waste into biogas and digestate.
Biogas production occurs within sealed reactors, which vary in design and size based on feedstock and site conditions. The resulting biogas and digestate offer valuable energy and material resources, contributing to sustainable waste management practices.
Geothermal Energy
Geothermal energy refers to the natural heat originating from the earth’s interior, which can be harnessed to produce electricity and heat structures. Beneath the earth’s crust lies a layer of hot, molten rock known as magma. The continuous decay of naturally occurring radioactive elements like uranium and potassium within this layer generates heat.
Remarkably, the heat within 10,000 meters (33,000 feet) of the earth’s surface holds 50,000 times more energy than the combined reserves of oil and natural gas worldwide. Geothermal resources are categorized into three types: pressurized zones, hot-rock zones, and hydrothermal convection zones. Presently, only the first type is being commercially exploited.
Natural manifestations of geothermal energy include hot springs, geysers, and lava fountains. In India, the Ministry of New and Renewable Energy has mapped geothermal resources, estimating a potential of 10 gigawatts (GW) of geothermal power.
Basic Types of Geothermal Heat Resource Extraction
- Hydrothermal Heat Source Hydrothermal heat sources involve the transfer of heat to the surface through water, which is replenished into the earth by precipitation or surface water bodies like rivers and lakes. This water is then heated by underlying hot rock layers resulting from seismic or volcanic activity. This process is evident in locations with hot springs such as Iceland, the Himalayas, and the Alps. Porous lithology allows water to flow freely, replenishing the system and transporting hot water to the surface.Â
- Deep/Enhanced Geothermal System Deep/enhanced geothermal systems entail drilling deep boreholes to access the hot basal rock layers. Water is then injected into these boreholes to produce steam, which powers turbines for electricity generation. However, this method is less commonly used due to the high cost associated with accessing the deep basal rock bed.Â
Geothermal Resources in India:
Geothermal resources in India are found in both orogenic (mountain-building) and non-orogenic regions. The following are potential sites categorized by their geological regions:
Orogenic Regions:
- Himalayan Geothermal ProvinceÂ
- Naga-Lushai Geothermal ProvinceÂ
- Andaman-Nicobar Islands Geothermal ProvinceÂ
Non-Orogenic Regions:
- Cambay GrabenÂ
- Son-Narmada-Tapi GrabenÂ
- West CoastÂ
- Damodar ValleyÂ
- Mahanadi ValleyÂ
- Godavari ValleyÂ
Specific Potential Sites:
- Puga Valley (Jammu and Kashmir)Â
- Tattapani (Chhattisgarh)Â
- Godavari Basin Manikaran (Himachal Pradesh)Â
- Bakreshwar (West Bengal)Â
- Tuwa (Gujarat)Â
- Unai (Maharashtra)Â
- Jalgaon (Maharashtra)Â
Challenges of Geothermal Energy:
Infrastructure Development
In addition to resource scarcity, inadequate infrastructure hampers the widespread adoption of geothermal electricity. Geothermal energy serves as baseline power, requiring specialized equipment for drilling wells and constructing plants. The scarcity of trained personnel further complicates operations, with logistical challenges in transporting extracted energy to distant grids.
High Initial Investment
Despite its long-term benefits, geothermal energy extraction incurs substantial upfront costs. While production costs have declined, exploration and drilling expenses remain significant. Geothermal areas’ harsh geological conditions necessitate frequent equipment replacements, adding to operational costs.
Finite Resource Management
Contrary to assumptions, geothermal reserves are finite and require careful management. Water and steam extraction from wells must be balanced with reinjection to maintain pressure gradients. Failure to restore these gradients may deplete energy sources and pose geological risks like sinkhole formation.
Transmission Limitations
Geothermal plants must be proximal to reservoirs due to transmission constraints. However, prime geothermal sites are often rural, complicating electricity grid integration. Installing new power lines entails high expenses and logistical hurdles.
Environmental Considerations
While geothermal energy is cleaner than fossil fuels, excavation activities can release greenhouse gases. Though emissions are lower than those from fossil fuel plants, they contribute to environmental concerns. Furthermore, enhanced geothermal systems may induce seismic activity, necessitating cautious monitoring and mitigation strategies.
Sustainability Measures
Sustainable geothermal practices mandate reinjection of extracted fluid to maintain reservoir integrity. Long-term viability necessitates careful resource management and mitigation of adverse environmental impacts. Balancing benefits with risks is crucial for maximizing geothermal energy’s potential while ensuring environmental stewardship.
Operational Challenges
Construction activities may inadvertently release harmful gases from underground reservoirs. Effective containment and safe disposal mechanisms are imperative for addressing potential hazards and ensuring operational safety.
Fuel Cells
A fuel cell is an electrochemical device that converts chemical energy directly into electrical energy. Operating on the principle of a chemical reaction between a positively charged ion (hydrogen) and an oxidizing agent (oxygen), fuel cells offer a sustainable energy solution. Fuel cells exhibit diverse configurations, yet they commonly consist of a cathode, an anode, and an electrolyte enabling the movement of positively charged ions, such as hydrogen, between electrodes. Unlike batteries, fuel cells require a continuous supply of fuel to sustain operation, producing direct current (DC) electricity in the process.
How do Fuel Cells work?Â
Fuel cells share similarities with batteries, delivering continuous power without the need for recharging. As long as fuel is available, they generate electricity and heat. Typically composed of two electrodes encasing an electrolyte, fuel cells feature a negative electrode (anode) and a positive electrode (cathode). Hydrogen serves as fuel for the anode, while oxygen from the air interacts with the cathode. A catalyst at the anode triggers the breakdown of hydrogen molecules into protons and electrons, each following distinct paths. Electrons traverse an external circuit, facilitating the flow of electricity. Meanwhile, protons navigate through the electrolyte to the cathode, where they combine with oxygen and electrons to produce water and heat.
Advantages:
- Low Emissions: Fuel cells powered by pure hydrogen emit minimal pollutants, with stationary power plants producing less than one ounce of pollution per 1,000 kilowatt-hours of electricity.Â
- Noise Reduction: Fuel cells operate quietly, emitting around 60 decibels, similar to a regular conversation, due to their combustion-free operation and minimal moving parts.Â
- High Efficiency: Generating energy electrochemically, fuel cells achieve fuel-to-electricity efficiency of 40-50% using hydrocarbon fuels like natural gas.Â
- Energy Security: Hydrogen, a domestically producible resource, enhances energy security, reducing dependency on foreign oil imports and decentralized power generation.Â
- Constant Power Supply: Fuel cells provide continuous power, ensuring critical connections and uninterrupted power during weather events like hurricanes.Â
- Durability and Scalability: Fuel cells are durable, scalable, and modular, suitable for various applications from military operations to residential and commercial settings.Â
- Lightweight and Longevity: Portable fuel cells offer longer operational life and lighter weight compared to batteries, ensuring continuous energy production without the need for recharging.Â
Challenges:
- Hydrogen Extraction: Extracting hydrogen from water or fossil fuels demands substantial energy, which may exceed the energy obtained from hydrogen alone, posing cost and environmental challenges.Â
- Investment Requirement: The viability of hydrogen fuel cells requires significant investment in research, development, and infrastructure, necessitating political and financial commitment.Â
- Cost of Raw Materials: Fuel cells requiring precious metals like platinum and iridium as catalysts incur high initial costs, deterring widespread adoption.Â
- Regulatory Hurdles: Ambiguous regulatory frameworks hinder commercial deployment, affecting investment decisions and complicating cost assessments.Â
- Overall Cost: Hydrogen fuel cells currently cost more per unit of power compared to alternative energy sources like solar panels, impeding broad adoption.Â
- Hydrogen Storage: Challenges in hydrogen storage and transportation add to the overall cost and complexity of fuel cell technology.Â
- Infrastructure and Safety Concerns: Establishing refueling infrastructure and addressing safety concerns associated with hydrogen’s highly combustible nature present additional challenges to widespread adoption.Â
FAQs
1. What is solar energy, and how is it harnessed?
Solar energy is derived from the sun’s radiation. It is harnessed using photovoltaic cells or solar panels, which convert sunlight directly into electricity. Additionally, solar thermal systems use mirrors or lenses to concentrate sunlight to heat a fluid, which then produces steam to generate electricity through a turbine.
2. How does wind energy work, and what are its advantages?
Wind energy is generated by wind turbines, which capture the kinetic energy of the wind and convert it into electricity. The spinning blades of the turbine drive a generator, producing electricity. Its advantages include being abundant, renewable, and producing no greenhouse gas emissions during operation.
3. What are tidal and wave energies, and how do they differ?
Tidal energy is generated by harnessing the movement of tides caused by the gravitational pull of the moon and the sun. It typically involves building tidal barrages or turbines in coastal areas. Wave energy, on the other hand, captures the energy from ocean waves using devices such as oscillating water columns or point absorbers, which convert the up-and-down motion of waves into electricity.
4. How is geothermal energy extracted, and where is it commonly found?
Geothermal energy is derived from the heat stored beneath the Earth’s surface. It is extracted by drilling wells into hot reservoirs of steam or hot water. This steam or hot water is then used to generate electricity through a turbine connected to a generator. Geothermal energy is commonly found in regions with tectonic activity, such as along tectonic plate boundaries.
5. What are fuel cells and how do they contribute to renewable energy?
Fuel cells generate electricity through an electrochemical reaction between hydrogen and oxygen, with the only byproduct being water. They can use hydrogen produced from renewable sources, such as electrolysis powered by renewable electricity, making them a clean and sustainable energy option. Fuel cells can be used for various applications, including transportation and stationary power generation.
In case you still have your doubts, contact us on 9811333901.
For UPSC Prelims Resources, Click here
For Daily Updates and Study Material:
Join our Telegram Channel – Edukemy for IAS
- 1. Learn through Videos – here
- 2. Be Exam Ready by Practicing Daily MCQs – here
- 3. Daily Newsletter – Get all your Current Affairs Covered – here
- 4. Mains Answer Writing Practice – here